ABOVE: Spherical colonies of Vibrio splendidus bacteria Julia Schwartzman
Julia Schwartzman, a microbiologist at MIT, was flummoxed. She was studying the conditions under which bacteria cooperate using Vibrio splendidus 12B01, a strain of an algae-eating species common in ocean water. But even though she was performing what she describes as “the simplest experiment” she could think of—growing V. splendidus in a glass flask that contained large, complex sugars derived from algae—the growth curves for her colonies were off.
In culture, bacteria typically grow exponentially. And at first, that’s what Schwartzman saw. But then the growth rate “would go just crazy,” she says, going up and down erratically such that jagged peaks formed on her initially smooth curves. “Instead of this beautiful exponential growth curve, the results were looking terrible,” she tells The Scientist.
Frustrated, she decided to look at what was going on under the microscope. While she expected the bacteria to aggregate in haphazard clumps, V. splendidus had grown into neat, nearly perfect spheres. These bizarre colonies, described June 30 in Current Biology, turned out to be a previously unknown form of bacterial self-organization. And although these clustered cells share the same genes, Schwartzman and her colleagues found that they expressed them differently based on their location in the sphere, taking on starkly different roles in their miniature community.
“This is a really cool study. It highlights bacterial differentiation using an incredibly neat system. Bacterial differentiation is a really important biological process that we are just beginning to understand,” Josephine Chandler, a microbiologist at the University of Kansas who was not involved in the work, writes in an email to The Scientist.
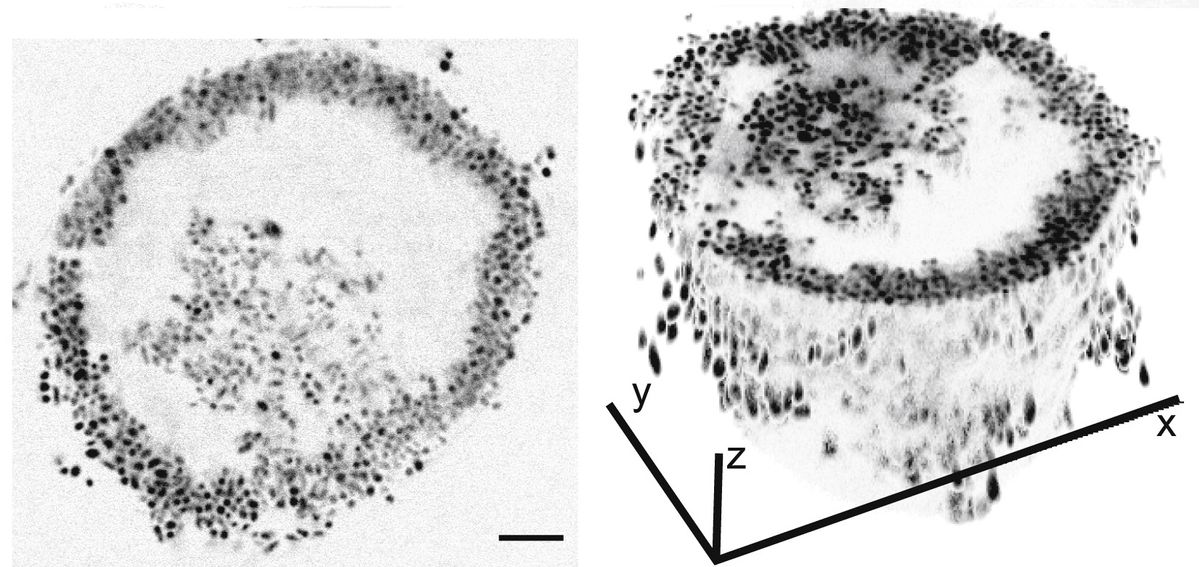
Although the spheres were unanticipated, Schwartzman says she had expected the bacteria to cooperate. That’s because the microbes would need to break down the fuel she provided to them—large polysaccharides—into tiny, digestible pieces. A solo bacterium could secrete sugar-busting enzymes into its watery environment, but then would have to hunt down the broken-down sugar wherever it may have drifted off to. Together, bacteria can break down and slurp up large sugars more effectively.
Typically, bacteria engage in this sort of cooperation by forming biofilms and other aggregates, in which they’re glued together by a dense extracellular matrix. In this form, they can share nutrients and genetic information. Indeed, Schwartzman had previously observed that V. splendidus clump up when suspended in liquid media, but the colonies that the bacteria formed this time around were much neater and more organized than she expected. By observing these colonies through a microscope, the researchers realized that as the cells divided and created a tight cluster and started to take on different properties depending on their location in the clump. Those along the margins moved very little, creating a static shell, while those in the core continued to move around. Both types of cells then continued to grow and divide until the interior cells eventually burst out through their external companions, leaving a hollow sphere. After the rupture, the authors speculate that the cells remaining in the shell die off while the escapees go on to form new colonies, pointing out that this bursting behavior, which the scientists described as a three-stage process, is similar to how fungi and other organisms propagate.
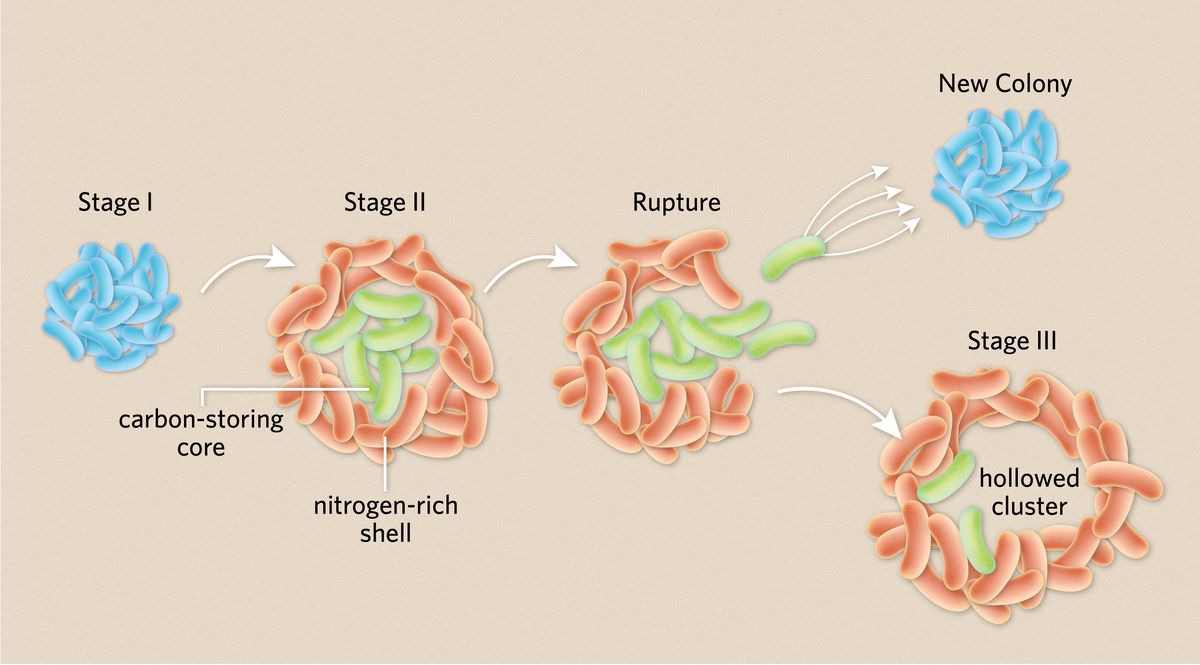
This alternating growing and bursting behavior likely led to the “bouncing around” Schwartzman saw in the growth curves, she says. As the cells formed spheres, the number of bacteria increased. Then, growth suddenly paused after the colonies ruptured, she speculates.
After observing the two phenotypes that create such structured colonies, Schwartzman employed a battery of techniques to understand how they emerge. RNA sequencing revealed that the core and shell populations were transcriptionally different. The cells in the shell were pumping out filamentous protein structures called type 4 pilli that can expand and retract—Schwartzman likens them to “active Velcro.” This is likely how these outer cells stick to each other and keep the structure contained.
Meanwhile, the cells in the core activated genes for making lipids, which Schwartzman says was likely a way of stockpiling carbon that the colony could use as it grew.
The researchers also wanted to understand how the colony was sharing resources. Using a technique called nanoSIM, they grew the bacteria in the presence of a heavy nitrogen isotope and measured how well the cells assimilated this nitrogen. They found that the shell took up more nitrogen than the core, meaning that there was likely a nutrient gradient across the colony. Other nutrients such as oxygen were likely also less available to cells in the core.
“The sticky shell is important to hold all the cells together in a neighborhood so that they can get resources and cooperate,” Schwartzman explains. But this peaceful coexistence doesn’t last. The cells in the middle are taking in more of the resources—mainly carbon—that are necessary for growth and future propagation. Meanwhile, these core cells are also being deprived of other vital nutrients. So eventually, they burst out and form their own colonies.
“We think it’s a really nice example of what would be called a division of labor,” says Schwartzman—a phenomenon rare in nature, the researchers say, even among bacteria.
In the wild, they explain, these bacteria are likely using the division of labor strategy to take advantage of temporary feasts. V. splendidus spend the majority of their lives as single cells floating around in ocean water looking for food, the researchers say. Once they find an abundant source, the divison of labor strategy allows them to break down and store complex sugars quickly and efficiently.
See: “Cancer-Like Slime Mold Hints at Multicellularity’s Origins”
Chandler speculates that this strategy could also be found elsewhere in nature. “It would not surprise me at all if it was found to be common to almost all types of bacteria,” she says.
Like this article? You might also enjoy our bimonthly microbiology newsletter, which is filled with stories like it. You can sign up for free here. |